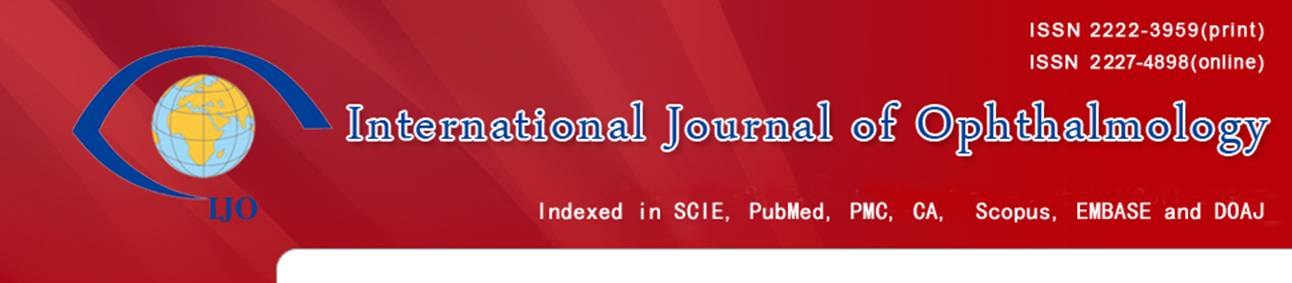
·Review Article·
Potential
applications of artemisinins in ocular diseases
Bing-Wen Lu, Li-Ke Xie
Department of Ophthalmology, Eye
Hospital, China Academy of Chinese Medical Sciences, Beijing 100400, China
Correspondence to: Li-Ke Xie. Department of
Ophthalmology, Eye Hospital, China Academy of Chinese Medical Sciences, Beijing
100400, China. bjxielike@sina.com
Received: 2018-08-28
Accepted: 2019-04-17
Abstract
Artemisinin, also named qinghaosu, is a
family of sesquiterpene trioxane lactone originally derived from the sweet
wormwood plant (Artemisia annua), which is a traditional Chinese herb
that has been universally used as anti-malarial agents for many years. Evidence
has accumulated during the past few years which demonstrated the protective
effects of artemisinin and its derivatives (artemisinins) in several other
diseases beyond malaria, including cancers, autoimmune disorders, inflammatory
diseases, viral and other parasite-related infections. Recently, this long-considered
anti-malarial agent has been proved to possess anti-oxidant, anti-inflammatory,
anti-apoptotic and anti-excitotoxic properties, which make it a potential
treatment option for the ocular environment. In this review, we first described
the overview of artemisinins, highlighting the activity of artemisinins to
other diseases beyond malaria and the mechanisms of these actions. We then
emphasized the main points of published results of using artemisinins in
targeting ocular disorders, including uveitis, retinoblastoma, retinal
neurodegenerative diseases and ocular neovascularization. To conclude, we
believe that artemisinins could also be used as a promising therapeutic drug
for ocular diseases, especially retinal vascular diseases in the near future.
KEYWORDS: artemisinins; uveitis;
retinoblastoma; retinal neurodegenerative diseases; ocular neovascularization
DOI:10.18240/ijo.2019.11.20
Citation: Lu
BW, Xie LK. Potential applications of artemisinins in ocular diseases. Int J
Ophthalmol 2019;12(11):1793-1800
INTRODUCTION
Artemisinin and its derivatives
(artemisinins) are isolated from the one ancient Chinese plant Artemisia
annua (more commonly known as sweet wormwood), which have been used in
traditional Chinese medicine (TCM) for fevers and chills[1].
Following the isolation of the active agent by Dr. You-You Tu’s group from the
Chinese Academy of TCM in the 1970s, artemisinin-based combination therapies
have joined the currently established standard treatments of malarial parasites
around the world[2-4].
Interestingly, abundant evidences have also demonstrated that artemisinins
might also be of therapeutic value for many other diseases beyond malaria,
including cancers, autoimmune disorders, inflammatory diseases as well as other
infectious conditions[5]. Recently, many
ophthalmologists and researchers have also showed their great interest in
artemisinins, especially artesunate and dihydroartemisinin (DHA) and their
potential protective effects on ocular disorders. Herein, we present an
overview of research advances of artemisinins as potential therapeutic methods
for ocular diseases, including uveitis, retinoblastoma, retinal neurodegenerative
diseases, especially ocular neovascularization (NV). In this review, we also
emphasize some important points regarding the potential applications of
artemisinins in ocular disorders to provide a platform for additional study.
OVERVIEW OF ARTEMISININS
History and Origins The medicinal herb Artemisia
annua was first recognized by one Chinese physician, Hong Ge (born in the
year 283) for its fever-reducing properties[6].
Led by the Chinese project 523 in
the 1970s, Dr. You-You Tu’s group first successfully isolated artemisinin, a
non-toxic extract of Artemisia annua, identified the active component of
this extract in 1972 and further identified its stereostucture (sesquiterpene
lactones) in 1975[1]. In the 1980-90s, further
studies conducted in humans confirmed the recognition of artemisinin-based
combination therapies as the first-line option to treat malaria[2-4]. This novel anti-malaria therapy
has been used universally with great efficacy and safety for a long time and
helped Dr. You-You Tu win the 2015 Nobel Prize in Physiology or Medicine for
her outstanding achievements[7].
Chemical and Pharmacological Characteristics It was Dr. You-You Tu who first
clarified the molecule extracted from the herbaceous plant Artemisia annu
to be a sesquiterpene lactone endoperoxide by using the combined method of mass
spectroscopy, spectrophotometry, X-ray crystallography and polyarithmetic
analysis[8]. Those clinically important
artemisinins include artesunate, artemether, arteether, and DHA (Figure 1),
discovered and developed in 1986[1]. Among which
artesunate is the most important analog, which shows a more favorable
pharmacological profile because of its greater water-solubility and high oral
bioavailability due to the additional hemisuccinate group[9].
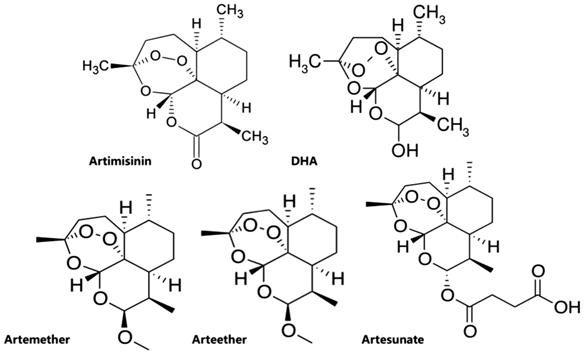
Figure 1 Chemical structures of
artemisinins.
Beyond Malaria: Activity of
Artemisinins to Other Diseases While the efficacy and low toxicity
of artemisinins to treat malaria is well-recognized around the world, they have
currently been reported to have a great therapeutic value beyond malaria[10]. These capacities include protective functions in
non-malaria parasitic infections[11-14],
anti-viral[15-17] and
anti-fungal properties[18-19],
anti-cancer functions[20-24],
as well as anti-inflammatory[25-27]
and anti-allergic effects[28-29]
(Figure 2). Recent results further indicated that artemisinins might also
reduce glucose, thus exerting a protective effect on diabetes mellitus[30].
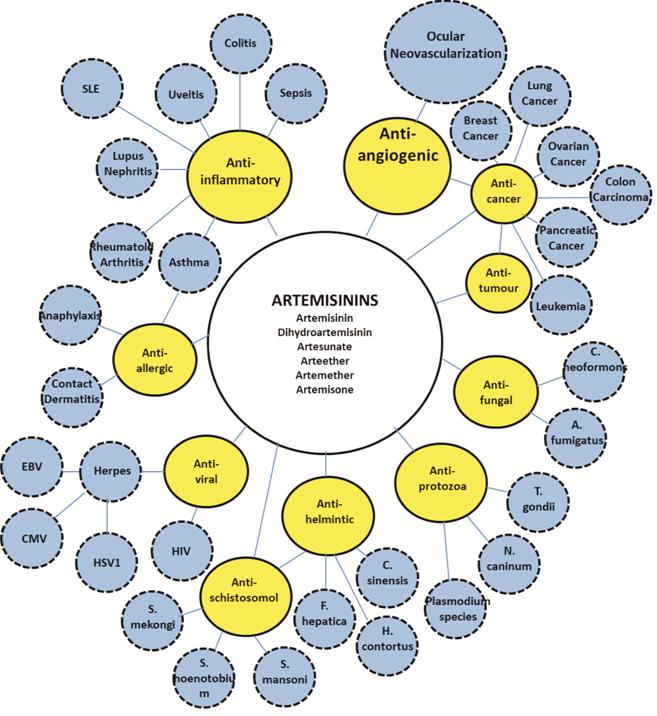
Figure 2 Various biological
activities of artemisinins and potential applications in different diseases
(Bubble map).
Mechanisms of Actions of
Artemisinins Although artemisinins are long known
and effectively used as anti-malaria drugs, their specific biological action is
poorly identified and understood. Current in vivo and in vitro studies
have proposed numerous possible mechanisms of the actions, which include 1)
oxidative stress, 2) induction of apoptosis[31],
3) inhibition of angiogenesis[32-33],
4) arrest of cell cycle at G0/G1[34]
(Figure 3). As a matter of fact, these functional pathways may overlap in a
number of ways.
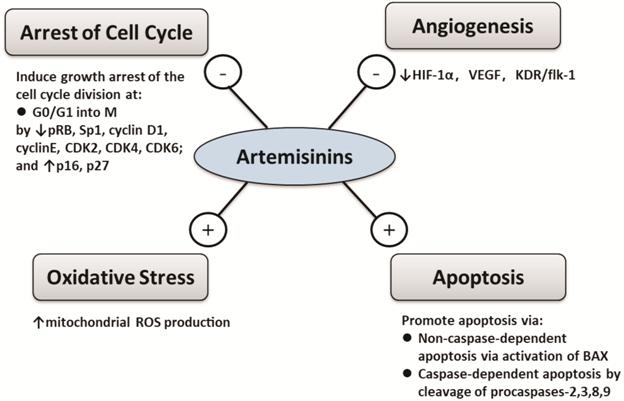
Figure 3 Overview of mechanisms of
actions of artemisinins - indicates inhibition and +
indicates activation.
Oxidative Stress Reactive oxygen species (ROS) are
the natural byproduct of aerobic metabolism, whose levels can dramatically
elevate during times of environmental stress. Studies in various tumor cell
lines have proved ROS to have an important role in artemisinins-induced
apoptosis[31]. These studies covered
neuroblastoma[32], breast cancer[33], T-cell lymphoma[34],
embryonal rhabdomyosarcoma cells[35], and
glioblastoma[36]. In a recent study on human hepatocellular
carcinoma cells, artesunate was shown to be able to induce ROS-dependent
apoptosis via Bax-mediated intrinsic pathway[37].
Similarly, DHA was shown to alleviate oxidative stress in bleomycin-induced
pulmonary fibrosis[38].
Induction of Apoptosis Apoptosis, or programmed cell death,
is a regulated cellular suicide mechanism involving the degradation of cellular
components, which can be initiated via the intrinsic pathway and the
extrinsic pathway[39]. Artemisinins could trigger
apoptotic cell death through both pathways[40-41]. In human colon cancer cell line (HT29), B-cell
lymphoma 2 associated X protein (BAX) was proved to be activated by
artemisinins, inducing the release of cytochrome C, which led to apoptosis in
cancer cells[42]. In human prostate cancer cell
line (DU145), cleavage of procaspases 3 and 9 was found to be induced by artesunate,
inducing the release of cytochrome C and the subsequent caspase-dependent
apoptosis[43]. In human breast cancer cell line
(MCF-7), apoptosis was also induced via a caspase-related mechanism
under the effect of a semi-synthetic derivative of artemisinin[44].
Inhibition of Angiogenesis Various models have accumulated
mounting evidences, demonstrating the involvement of inhibiting aberrant angiogenesis
in the actions of artemisinins[45-46].
In mouse embryonic stem cells, artemisinin was shown to be able to reduce the
levels of hypoxia inducible factor (HIF)-1α and vascular endothelial growth factor
(VEGF), suggesting the mechanism of artemisinin might involve the inhibition of
angiogenesis[47]. Artemisinin was also found to
be able to significantly reduce lymph-angiogenesis via downregulating
the expression of VEGF-C in C57BL/6 mouse Lewis lung carcinoma model[48]. Similarly, in a rat glioma model, artemisinins were
shown to have the effect of reducing VEGF and angiogenesis[49].
Moreover, artesunate was proved to be able to suppress osteoclastogenesis and
aberrant angiogenesis, thus attenuating anterior cruciate ligament transection
(ACLT)-induced osteoarthritis[50].
Arrest of Cell Cycle at G0/G1 Artemisinins have been shown by accumulating current
studies to have the potential application in cancer drug development for its
action on inducing growth arrest at various stages of cell division cycle[51-53]. In prostate cancer cells
(LNCaP), phosphorylated retinoblastoma protein (pRB), a mediator cooperating
with E2F
transcription factors and cyclin-dependent kinases (CDKs) to push forward the
cell cycle progression through G1 into S phase was shown to be
ablated by artemisinin, inducig G1 cell cycle arrest, thus
inhibiting cell division[54]. Willoughby et al[55] has also demonstrated that artemisinin could disrupt
specificity protein 1 (Sp1) transcription factor from binding to CDK4 promoter
and inhibiting CDK4 gene expression, thus blocking prostate cancer growth and
cell cycle progression. Wu et al[56] have
further proved the growth inhibition effect of artemisinin in nasopharyngeal
carcinoma cell lines by suppressing the level of cyclin D1, cyclin E, CDK2,
CDK4, CDK6 and upregulating the inhibitors of cell cycle division (p16, p27).
POTENTIAL APPLICATION IN OCULAR DISEASES
Recently, many ophthalmologists and
researchers have noticed the potential protective effects of artemisinins on
ocular disorders. Recent findings have shed light on the potential applications
of artemisinins as promising therapeutic agents in ocular diseases. In this
review, we are going to highlight the main points of published results of using
artemisinins in targeting ocular disorders.
Uveitis Uveitis is the inflammation of the
uvea whereas the anti-inflammatory effects of artemisinins have already been
recognized in the past few decades[57].
Artesunate has been reported by Li et al[58]
to have a protective effect on sepsis mouse model by decreasing serum endotoxin
release and toll-like receptors (TLR)4, TLR9 expressions, also suppressing
nuclear factor-kappa B (NF-κB) activation. Xu et al[59]
also reported that in human rheumatoid arthritis fibroblast-like
synoviocytes, artesunate was able to inhibit TNF-α expression and decrease the
secretion of pro-inflammatory cytokines. Based on those experimental results,
the question of if artesunate could reduce the release of inflammatory
cytokines in some type of inflammatory ocular diseases was raised and further
investigated. Wang et al[60] studied the
protective effect of artesunate by using endotoxin-induced uveitis (EIU) rat
model, which has been generally considered as an experimental model for human
uveitis[61]. In their study, artesunate of three
concentrations (1, 10, 100 mg/kg) were intravenously injected in male
Long-Evans rats whereas prednisolone (10 mg/kg) was used as positive control
and their results showed that artesunate (10 mg/kg and 100 mg/kg) could
suppress infiltrating cells and protein concentration in the aqueous humor, suggesting
that artesunate treatment could suppress the inflammation of EIU by inhibiting
the production of inflammatory mediators[60].
More future studies will be needed to clearly define the specific cellular
mechanisms of the therapeutic effects. The role of artemisinins in modulating
ocular inflammatory responses might be of great interest in the future.
Retinoblastoma In recent years, artemisinins have
been shown to exert protective effects in various types of cancer[62-66]. Retinoblastoma (RB) is an eye
cancer, which is most common among children[67].
Zhao et al[68] tested the anti-neoplastic activity
of artesunate against RB to see whether artesunate might be a good candidate to
treat RB. Using epithelial retina cell line as normal counterpart, the
cytotoxic activity and specificity of artesunate were analyzed in an RB cell
line, which showed a dose-dependent manner concerning the cytotoxic activity
specific to RB cells, with low toxicity in normal retina cells and high
cytotoxicity in RB cells[68]. Their results also
demonstrated that artesunate, even at low doses, could block the cell cycle
progression at the G1 phase[68].
Artesunate is practically suitable for long-term treatments with few
side-effects. Therefore, artesunate could be considered as a promising option
for RB treatment. Further randomized studies in vivo need to be done to
provide better insights regarding the efficacy as well as efficiency of the
novel treatment.
Retinal Neurodegenerative
Diseases Retinal neurodegeneration is a
retinopathy which consists in the deterioration of the retina caused by the
progressive death of its neuronal cells[69].
There are several reasons for retinal neurodegeneration, including age-related
macular degeneration (AMD), diabetic retinopathy (DR), and retinal artery or vein
occlusion[69]. Zeng et al[70] studied the neurogenic effects of artemisinin and
their findings indicated that artemisinin at low concentration could induce
neurite outgrowth as well as promote neuronal differentiation in PC12 cells.
Accordingly, Chong and Zheng[71] demonstrated that artemisinin was able to suppress
hydrogen peroxide (H2O2)-induced oxidative stress in D407
retinal pigment epithelium (RPE) cells, which are first damaged in retinal
diseases owning to their critical support functions for photoreceptors. The
findings of Yan et al[72] also
demonstrated that artemisinin could prevent RPE cells from oxidative stress via
the MAPK/CREB pathway.
These recent results all shed light
on the promising therapeutic value of artemisinin as a candidate drug for the
treatment of many retinal neurodegenerative disorders. Though, its specific
effects on the retinal neuronal cells need to be further explored.
Ocular Neovascularization Ocular NV is one of the major causes
of blindness among ocular disorders. Substantial evidences have demonstrated
that VEGF played an essential part in its pathogenesis[73].
Currently for the treatment of ocular NV, anti-VEGF agents such as ranibizumab
and bevacizumab are widely used[74-75].
However, these drugs both have a large molecular weight and resistance to these
drugs is usually seen in approximately 20%-30% ocular NV patients[76]. Moreover, because of the short aqueous half-life,
the recurrence rate is high after anti-VEGF treatments which may also increase
the risk of endophthalmitis owning to frequent intravitreal injections[77]. Abundant studies have already demonstrated the
anti-angiogenic effects of artemisinins in tumors[78].
The known mechanisms of artemisinins in inhibiting angiogenesis include
downregulating several growth factors, inducing apoptosis of vascular
endothelial cells, upregulating angiogenesis inhibitors, depleting the levels
of the flt-1 and KDR/flk-1-receptors[79-80].
In human umbilical vein endothelial cell (HUVEC) lines, artesunate was shown to
inhibit angiogenesis through downregulating the levels of the VEGF receptors[81]. Similar protective effects were also investigated in
lymphatic endothelial cells and Lewis lung carcinoma cells with the treatment
of DHA[82]. In the science of ophthalmology,
Cheng et al[83] demonstrated that
artesunate could inhibit corneal NV by inducing ROS-dependent apoptosis in
animal models. Their results suggested that artesunate could markedly inhibit
angiogenesis by specifically inducing apoptosis via an
iron/ROS-dependent p38 MAPK-mitochondrial pathway in vascular endothelial cells[83]. Zong et al[84]
further investigated the use of artesunate in retinal NV and found that retinal
NV could be remarkably inhibited under the effect of artesunate via
downregulating the expression of VEGFR2, and PDGFR. Compared to bevacizumab,
artesunate could remarkably inhibit retinal NV in rabbits with more durable
efficacy. These two published animal evidences indicated the potential role of
artesunate as a promising drug candidate to manage ocular NVs. As a
newly-discovered anti-angiogenesis drug, artemisinins are worthwhile to be
further explored due to a host of advantages.
Compared to the currently used
anti-VEGF drugs, the advantages of artesunate are as follows: 1) Small molecule
size: artesunate is a 384 Da molecule less than one-hundredth the size of
bevacizumab (149 kDa); 2) Safety and low toxicity: artesunate has been widely
used for many years as anti-malarial agents, with few adverse side effects and
proven safety records; 3) Multi-targets: artesunate was proved to possess not
only anti-angiogenetic effects targeting multi-growth factors (VEGF, FGF, HIF-1ɑ,
and Ang-1), but also anti-inflammatory and anti-apoptotic effects.
Thus, we postulate that artesunate
might be a potential novel treatment option for retinal vascular diseases such
as AMD, DR, retinal artery or vein occlusion, especially when given
intravitreously or being formulated into eye drops.
LIMITATIONS OF ARTEMISININS
The present studies of artemisinins
have several limitations. While applying artemisinins for treatments beyond
malaria, different research groups have reported inconsistent effective doses
even for similar cell lines or animal models. Progress for further clinical
trials could be hampered for the lack of a concerted effort to confirm the efficacies
of artemisinins in different models. Another limitation is the lack of acute
and chronic toxicological studies for acute as well as chronic exposure to
artemisinin in ocular diseases, which is necessary for future application in
ocular diseases.
CONCLUSION
To date, researches on artemisinins
and its applications in ocular diseases are still limited, and much more will
need to be studied. Further understanding of the protective activities of
artemisinins beyond malaria might lead to improved treatments for ocular
disorders.
In this review, we summarized recent
studies on artemisinins in treating ocular diseases and we believe that this
anti-malaria agent could also be used as a promising therapeutic drug for
ocular diseases, especially retinal vascular diseases.
ACKNOWLEDGEMENTS
Authors’ contributions: Lu BW wrote the manuscript; Xie LK
participated discussion and provided suggestion.
Conflicts of Interest: Lu BW, None; Xie LK, None.
REFERENCES
1 Tu Y. The discovery of artemisinin (qinghaosu)
and gifts from Chinese medicine. Nat Med 2011;17(10):1217-1220.
https://doi.org/10.1038/nm.2471
PMid:21989013
|
|
2 Song J, Socheat D, Tan B, Seila S, Xu Y, Ou
F, Sokunthea S, Sophorn L, Zhou C, Deng C, Wang Q, Li G. Randomized trials of
artemisinin-piperaquine, dihydroartemisinin-piperaquine phosphate and
artemether-lumefantrine for the treatment of multi-drug resistant falciparum
malaria in Cambodia-Thailand border area. Malar J 2011;10:231.
https://doi.org/10.1186/1475-2875-10-231
PMid:21827706 PMCid:PMC3169515
|
|
|
3 Makowiecki M, Bednarska A, Paciorek M,
Kowalska J, Skrzat-Klapaczyńska A, Puła J, Sosińska-Bryła I, Krogulec D,
Raczyńska J, Hackiewicz M, Stengiel J, Bursa D, Pihowicz A, Horban A.
Usefulness of SOFA score and artesunate-based treatment in severe malaria - a
single center study. Przegl Epidemiol 2018;72(2):215-221.
|
|
|
4 Mace KE, Arguin PM, Tan KR. Malaria
surveillance-United States, 2015. MMWR Surveill Summ 2018;67(7):1-28.
https://doi.org/10.15585/mmwr.ss6707a1
PMid:29723168 PMCid:PMC5933858
|
|
|
5 Raffetin A, Bruneel F, Roussel C,
Thellier M, Buffet P, Caumes E, Jauréguiberry S. Use of artesunate in
non-malarial indications. Médecine Et Maladies Infect 2018;48(4):238-249.
https://doi.org/10.1016/j.medmal.2018.01.004
PMid:29422423
|
|
|
6 van Agtmael MA, Eggelte TA, van Boxtel
CJ. Artemisinin drugs in the treatment of malaria: from medicinal herb to
registered medication. Trends Pharmacol Sci 1999;20(5):199-205.
https://doi.org/10.1016/S0165-6147(99)01302-4
|
|
|
7 Chen WJ. Honoring antiparasitics: the
2015 Nobel prize in physiology or medicine. Biomed J 2016;39(2):93-97.
https://doi.org/10.1016/j.bj.2016.04.002
PMid:27372164 PMCid:PMC6139675
|
|
|
8 Stringham RW, Moore GL, Teager DS, Yue
TY. Analysis and isolation of potential artemisinin precursors from waste
streams of Artemisia annua extraction. ACS Omega 2018;3(7):7803-7808.
https://doi.org/10.1021/acsomega.8b00974
PMid:30087924 PMCid:PMC6068693
|
|
|
9 Pinheiro LCS, Feitosa LM, Silveira FFD,
Boechat N. Current antimalarial therapies and advances in the development of
semi-synthetic artemisinin derivatives. An Acad Bras Cienc 2018;90(1 Suppl
2):1251-1271.
https://doi.org/10.1590/0001-3765201820170830
PMid:29873667
|
|
|
10 Loo CS, Lam NS, Yu D, Su XZ, Lu F.
Artemisinin and its derivatives in treating protozoan infections beyond
malaria. Pharmacol Res 2017;117:192-217.
https://doi.org/10.1016/j.phrs.2016.11.012
PMid:27867026 PMCid:PMC5316320
|
|
|
11 Nibret E, Wink M. Volatile components of
four Ethiopian Artemisia species extracts and their in vitro antitrypanosomal
and cytotoxic activities. Phytomedicine 2010;17(5):369-374.
https://doi.org/10.1016/j.phymed.2009.07.016
PMid:19683909
|
|
|
12 Hencken CP, Jones-Brando L, Bordón C,
Stohler R, Mott BT, Yolken R, Posner GH, Woodard LE. Thiazole, oxadiazole,
and carboxamide derivatives of artemisinin are highly selective and potent
inhibitors of Toxoplasma gondii. J Med Chem 2010;53(9):3594-3601.
https://doi.org/10.1021/jm901857d
PMid:20373807 PMCid:PMC2865576
|
|
|
13 Singh C, Kanchan R, Chaudhary S, Puri SK.
Linker-based hemisuccinate derivatives of artemisinin: synthesis and
antimalarial assessment against multidrug-resistant Plasmodium yoelii
nigeriensis in mice. J Med Chem 2012;55(3):1117-1126.
https://doi.org/10.1021/jm2010699
PMid:22216834
|
|
|
14 Ferreira JF, Peaden P, Keiser J. In
vitro trematocidal effects of crude alcoholic extracts of Artemisia annua, A.
Absinthium, Asimina triloba, and Fumaria officinalis: trematocidal plant alcoholic
extracts. Parasitol Res 2011;109(6):1585-1592.
https://doi.org/10.1007/s00436-011-2418-0
PMid:21562762
|
|
|
15 Fröhlich T, Reiter C, Saeed MEM, Hutterer
C, Hahn F, Leidenberger M, Friedrich O, Kappes B, Marschall M, Efferth T,
Tsogoeva SB. Synthesis of thymoquinone-artemisinin hybrids: new potent
antileukemia, antiviral, and antimalarial agents. ACS Med Chem Lett
2018;9(6):534-539.
https://doi.org/10.1021/acsmedchemlett.7b00412
PMid:29937978 PMCid:PMC6004568
|
|
|
16 Parvez MK, Arbab AH, Al-Dosari MS,
Al-Rehaily AJ. Antiviral natural products against chronic hepatitis B: recent
developments. Curr Pharm Des 2016;22(3):286-293.
https://doi.org/10.2174/1381612822666151112152733
PMid:26561057
|
|
|
17 Jana S, Iram S, Thomas J, Hayat MQ, Pannecouque
C, Dehaen W. Application of the triazolization reaction to afford
dihydroartemisinin derivatives with anti-HIV activity. Molecules
2017;22(2):E303.
https://doi.org/10.3390/molecules22020303
PMid:28218680 PMCid:PMC6155659
|
|
|
18 Chang CC, Sorrell TC, Chen SC. Pulmonary
cryptococcosis. Semin Respir Crit Care Med 2015;36(5):681-691.
https://doi.org/10.1055/s-0035-1562895
PMid:26398535
|
|
|
19 Gautam P, Upadhyay SK, Hassan W, Madan
T, Sirdeshmukh R, Sundaram CS, Gade WN, Basir SF, Singh Y, Sarma PU.
Transcriptomic and proteomic profile of Aspergillus fumigatus on exposure to
artemisinin. Mycopathologia 2011;172(5):331-346.
https://doi.org/10.1007/s11046-011-9445-3
PMid:21755315
|
|
|
20 Jamalzadeh L, Ghafoori H, Aghamaali M, Sariri
R. Induction of apoptosis in human breast cancer MCF-7 cells by a
semi-synthetic derivative of artemisinin: a caspase-related mechanism. Iran J
Biotechnol 2017;15(3):157-165.
https://doi.org/10.15171/ijb.1567
PMid:29845064 PMCid:PMC5811062
|
|
|
21 Li S, Li G, Yang X, Meng Q, Yuan S, He
Y, Sun D. Design, synthesis and biological evaluation of artemisinin
derivatives containing fluorine atoms as anticancer agents. Bioorg Med Chem
Lett 2018;28(13):2275-2278.
https://doi.org/10.1016/j.bmcl.2018.05.035
PMid:29789258
|
|
|
22 Yu H, Hou Z, Tian Y, Mou Y, Guo C.
Design, synthesis, cytotoxicity and mechanism of novel dihydroartemisinin-coumarin
hybrids as potential anti-cancer agents. Eur J Med Chem 2018;151:434-449.
https://doi.org/10.1016/j.ejmech.2018.04.005
PMid:29649740
|
|
|
23 Våtsveen TK, Myhre MR, Steen CB, Wälchli
S, Lingjærde OC, Bai B, Dillard P, Theodossiou TA, Holien T, Sundan A,
Inderberg EM, Smeland EB, Myklebust JH, Oksvold MP. Artesunate shows potent
anti-tumor activity in B-cell lymphoma. J Hematol Oncol 2018;11(1):23.
https://doi.org/10.1186/s13045-018-0561-0
PMid:29458389 PMCid:PMC5819282
|
|
|
24 Deeken JF, Wang H, Hartley M, Cheema AK,
Smaglo B, Hwang JJ, He AR, Weiner LM, Marshall JL, Giaccone G, Liu S, Luecht
J, Spiegel JY, Pishvaian MJ. A phase I study of intravenous artesunate in
patients with advanced solid tumor malignancies. Cancer Chemother Pharmacol
2018;81(3):587-596.
https://doi.org/10.1007/s00280-018-3533-8
PMid:29392450
|
|
|
25 Mu X, Wang C. Artemisinins-a promising
new treatment for systemic lupus erythematosus: a descriptive review. Curr
Rheumatol Rep 2018;20(9):55.
https://doi.org/10.1007/s11926-018-0764-y
PMid:30056574
|
|
|
26 Kuang M, Cen Y, Qin R, Shang S, Zhai Z, Liu
C, Pan X, Zhou H. Artesunate attenuates pro-inflammatory cytokine release
from macrophages by inhibiting TLR4-mediated autophagic activation via the
TRAF6-Beclin1-PI3KC3 pathway. Cell Physiol Biochem 2018;47(2): 475-488.
https://doi.org/10.1159/000489982
PMid:29794440
|
|
|
27 Jiao J, Yang Y, Liu M, Li J, Cui Y, Yin
S, Tao J. Artemisinin and Artemisia annua leaves alleviate Eimeria tenella infection
by facilitating apoptosis of host cells and suppressing inflammatory
response. Vet Parasitol 2018;254:172-177.
https://doi.org/10.1016/j.vetpar.2018.03.017
PMid:29657004
|
|
|
28 Deng Y, Liu Z, Geng Y. Anti-allergic
effect of Artemisia extract in rats. Exp Ther Med 2016;12(2):1130-1134.
https://doi.org/10.3892/etm.2016.3361
PMid:27446332 PMCid:PMC4950231
|
|
|
29 Li J, Wang B, Luo Y, Bian Y, Wang R.
Effect of artemisinin and neurectomy of pterygoid canal in ovalbumin-induced
allergic rhinitis mouse model. Allergy Asthma Clin Immunol 2018;14:22.
https://doi.org/10.1186/s13223-018-0249-6
PMid:29991950 PMCid:PMC5994650
|
|
|
30 Li J, Casteels T, Frogne T, et al.
Artemisinins target GABAA receptor signaling and impair α cell identity. Cell
2017;168(1-2):86-100.e15.
https://doi.org/10.1016/j.cell.2016.11.010
PMid:27916275 PMCid:PMC5236063
|
|
|
31 Tsuda K, Miyamoto L, Hamano S, Morimoto
Y, Kangawa Y, Fukue C, Kagawa Y, Horinouchi Y, Xu W, Ikeda Y, Tamaki T, Tsuchiya
K. Mechanisms of the pH- and oxygen-dependent oxidation activities of
artesunate. Biol Pharm Bull 2018;41(4):555-563.
https://doi.org/10.1248/bpb.b17-00855
PMid:29607928
|
|
|
32 Michaelis M, Kleinschmidt MC, Barth S,
Rothweiler F, Geiler J, Breitling R, Mayer B, Deubzer H, Witt O, Kreuter J,
Doerr HW, Cinatl J, Cinatl J Jr. Anti-cancer effects of artesunate in a panel
of chemoresistant neuroblastoma cell lines. Biochem Pharmacol
2010;79(2):130-136.
https://doi.org/10.1016/j.bcp.2009.08.013
PMid:19698702
|
|
|
33 Hamacher-Brady A, Stein HA, Turschner S,
Toegel I, Mora R, Jennewein N, Efferth T, Eils R, Brady NR. Artesunate
activates mitochondrial apoptosis in breast cancer cells via iron-catalyzed
lysosomal reactive oxygen species production. J Biol Chem
2011;286(8):6587-6601.
https://doi.org/10.1074/jbc.M110.210047
PMid:21149439 PMCid:PMC3057810
|
|
|
34 Wang Q, Wu S, Zhao X, Zhao C, Zhao H,
Huo L. Mechanisms of dihydroartemisinin and
dihydroartemisinin/holotransferrin cytotoxicity in T-cell lymphoma cells.
PLoS One 2015;10(10):e0137331.
https://doi.org/10.1371/journal.pone.0137331
PMid:26502166 PMCid:PMC4621048
|
|
|
35 Beccafico S, Morozzi G, Marchetti MC,
Riccardi C, Sidoni A, Donato R, Sorci G. Artesunate induces ROS- and p38
MAPK-mediated apoptosis and counteracts tumor growth in vivo in embryonal
rhabdomyosarcoma cells. Carcinogenesis 2015;36(9):1071-1083.
https://doi.org/10.1093/carcin/bgv098
PMid:26153023
|
|
|
36 Berte N, Lokan S, Eich M, Kim E, Kaina
B. Artesunate enhances the therapeutic response of glioma cells to temozolomide
by inhibition of homologous recombination and senescence. Oncotarget
2016;7(41):67235-67250.
https://doi.org/10.18632/oncotarget.11972
PMid:27626497 PMCid:PMC5341871
|
|
|
37 Pang Y, Qin G, Wu L, Wang X, Chen T.
Artesunate induces ROS-dependent apoptosis via a Bax-mediated intrinsic
pathway in Huh-7 and Hep3B cells. Exp Cell Res 2016;347(2):251-260.
https://doi.org/10.1016/j.yexcr.2016.06.012
PMid:27327234
|
|
|
38 Yang DX, Qiu J, Zhou HH, Yu Y, Zhou DL,
Xu Y, Zhu MZ, Ge XP, Li JM, Lv CJ, Zhang HQ, Yuan WD. Dihydroartemisinin
alleviates oxidative stress in bleomycin-induced pulmonary fibrosis. Life Sci
2018;205:176-183.
https://doi.org/10.1016/j.lfs.2018.05.022
PMid:29752961
|
|
|
39 Thomas RB, Joy S, Ajayan MS, Paulose CS.
Neuroprotective potential of Bacopa monnieri and Bacoside A against dopamine
receptor dysfunction in the cerebral cortex of neonatal hypoglycaemic rats.
Cell Mol Neurobiol 2013;33(8):1065-1074.
https://doi.org/10.1007/s10571-013-9973-0
PMid:23975094
|
|
|
40 Ho WE, Peh HY, Chan TK, Wong WS.
Artemisinins: pharmacological actions beyond anti-malarial. Pharmacol Ther
2014;142(1):126-139.
https://doi.org/10.1016/j.pharmthera.2013.12.001
PMid:24316259
|
|
|
41 Gunjan S, Sharma T, Yadav K, Chauhan BS,
Singh SK, Siddiqi MI, Tripathi R. Artemisinin derivatives and synthetic trioxane
trigger apoptotic cell death in asexual stages of Plasmodium. Front Cell
Infect Microbiol 2018;8:256.
https://doi.org/10.3389/fcimb.2018.00256
PMid:30094226 PMCid:PMC6070741
|
|
|
42 Riganti C, Doublier S, Viarisio D,
Miraglia E, Pescarmona G, Ghigo D, Bosia A. Artemisinin induces doxorubicin
resistance in human colon cancer cells via calcium-dependent activation of
HIF-1alpha and P-glycoprotein overexpression. Br J Pharmacol
2009;156(7):1054-1066.
https://doi.org/10.1111/j.1476-5381.2009.00117.x
PMid:19298255 PMCid:PMC2697684
|
|
|
43 Nakase I, Gallis B, Takatani-Nakase T,
Oh S, Lacoste E, Singh NP, Goodlett DR, Tanaka S, Futaki S, Lai H, Sasaki T.
Transferrin receptor-dependent cytotoxicity of artemisinin-transferrin
conjugates on prostate cancer cells and induction of apoptosis. Cancer Lett
2009;274(2):290-298.
https://doi.org/10.1016/j.canlet.2008.09.023
PMid:19006645
|
|
|
44 Zeng Z, Xu J, Zheng W. Artemisinin
protects PC12 cells against β-amyloid-induced apoptosis through activation of
the ERK1/2 signaling pathway. Redox Biol 2017;12:625-633.
https://doi.org/10.1016/j.redox.2017.04.003
PMid:28391183 PMCid:PMC5385605
|
|
|
45 Iyer AS, Chapoval SP. Neuroimmune semaphorin
4A in cancer angiogenesis and inflammation: a promoter or a suppressor? Int J
Mol Sci 2018;20(1):E124.
https://doi.org/10.3390/ijms20010124
PMid:30598022 PMCid:PMC6337608
|
|
|
46 Wei T, Liu J. Anti-angiogenic properties
of artemisinin derivatives (Review). Int J Mol Med 2017;40(4):972-978.
https://doi.org/10.3892/ijmm.2017.3085
PMid:28765885
|
|
|
47 Wartenberg M, Wolf S, Budde P, Grünheck
F, Acker H, Hescheler J, Wartenberg G, Sauer H. The antimalaria agent
artemisinin exerts antiangiogenic effects in mouse embryonic stem
cell-derived embryoid bodies. Lab Invest 2003;83(11):1647-1655.
https://doi.org/10.1097/01.LAB.0000098424.38003.FF
PMid:14615418
|
|
|
48 Wang J, Zhang B, Guo Y, Li G, Xie Q, Zhu
B, Gao J, Chen Z. Artemisinin inhibits tumor lymphangiogenesis by suppression
of vascular endothelial growth factor C. Pharmacology 2008;82(2):148-155.
https://doi.org/10.1159/000148261
PMid:18667841
|
|
|
49 Wu ZP, Gao CW, Wu YG, Zhu QS, Yan Chen,
Xin Liu, Chuen Liu. Inhibitive effect of artemether on tumor growth and
angiogenesis in the rat C6 orthotopic brain gliomas model. Integr Cancer Ther
2009;8(1):88-92.
https://doi.org/10.1177/1534735408330714
PMid:19174507
|
|
|
50 Zhao C, Liu Q, Wang K. Artesunate
attenuates ACLT-induced osteoarthritis by suppressing osteoclastogenesis and
aberrant angiogenesis. Biomed Pharmacother 2017;96:410-416.
https://doi.org/10.1016/j.biopha.2017.10.018
PMid:29031199
|
|
|
51 Abd El-Aal NF, Hamza RS, Magdy M.
Anti-angiogenic and anti-lymphangiogenic role of praziquantel and artemether
in experimental mansoniasis. Acta Parasitol 2017;62(4):708-716.
https://doi.org/10.1515/ap-2017-0085
PMid:29035850
|
|
|
52 Im E, Yeo C, Lee HJ, Lee EO. Dihydroartemisinin
induced caspase-dependent apoptosis through inhibiting the specificity
protein 1 pathway in hepatocellular carcinoma SK-Hep-1 cells. Life Sci
2018;192:286-292.
https://doi.org/10.1016/j.lfs.2017.11.008
PMid:29128513
|
|
|
53 Mungun HK, Li S, Zhang Y, Huang S, Jia
Z, Ding G, Zhang A. Dihydroartemisinin inhibits indoxyl sulfate (IS)-promoted
cell cycle progression in mesangial cells by targeting COX-2/mPGES-1/PGE2
cascade. Am J Transl Res 2018;10(2):422-431.
|
|
|
54 Steely AM, Willoughby JA Sr, Sundar SN,
Aivaliotis VI, Firestone GL. Artemisinin disrupts androgen responsiveness of
human prostate cancer cells by stimulating the 26S proteasome-mediated degradation
of the androgen receptor protein. Anticancer Drugs 2017;28(9):1018-1031.
https://doi.org/10.1097/CAD.0000000000000547
PMid:28708672
|
|
|
55 Willoughby JA Sr, Sundar SN, Cheung M,
Tin AS, Modiano J, Firestone GL. Artemisinin blocks prostate cancer growth
and cell cycle progression by disrupting Sp1 interactions with the
cyclin-dependent kinase-4 (CDK4) promoter and inhibiting CDK4 gene expression.
J Biol Chem 2009;284(4):2203-2213.
https://doi.org/10.1074/jbc.M804491200
PMid:19017637 PMCid:PMC2629082
|
|
|
56 Wu J, Hu D, Yang G, Zhou J, Yang C, Gao
Y, Zhu Z. Down-regulation of BMI-1 cooperates with artemisinin on growth
inhibition of nasopharyngeal carcinoma cells. J Cell Biochem
2011;112(7):1938-1948.
https://doi.org/10.1002/jcb.23114
PMid:21445878
|
|
|
57 Shirahama S, Kaburaki T, Nakahara H,
Tanaka R, Takamoto M, Fujino Y, Kawashima H, Aihara M. Epidemiology of
uveitis (2013-2015) and changes in the patterns of uveitis (2004-2015) in the
central Tokyo area: a retrospective study. BMC Ophthalmol 2018;18(1):189.
https://doi.org/10.1186/s12886-018-0871-6
PMid:30068311 PMCid:PMC6090933
|
|
|
58 Li B, Zhang R, Li J, Zhang L, Ding G,
Luo P, He S, Dong Y, Jiang W, Lu Y, Cao H, Zheng J, Zhou H. Antimalarial
artesunate protects sepsis model mice against heat-killed Escherichia coli
challenge by decreasing TLR4, TLR9 mRNA expressions and transcription factor
NF-kappa B activation. Int Immunopharmacol 2008;8(3):379-389.
https://doi.org/10.1016/j.intimp.2007.10.024
PMid:18279792
|
|
|
59 Xu H, He Y, Yang X, Liang L, Zhan Z, Ye
Y, Yang X, Lian F, Sun L. Anti-malarial agent artesunate inhibits TNF-alpha-induced
production of proinflammatory cytokines via inhibition of NF-kappaB and PI3
kinase/Akt signal pathway in human rheumatoid arthritis fibroblast-like
synoviocytes. Rheumatology (Oxford) 2007;46(6):920-926.
https://doi.org/10.1093/rheumatology/kem014
PMid:17314215
|
|
|
60 Wang XQ, Liu HL, Wang GB, Wu PF, Yan T,
Xie J, Tang Y, Sun LK, Li C. Effect of artesunate on endotoxin-induced
uveitis in rats. Invest Ophthalmol Vis Sci 2011;52(2):916-919.
https://doi.org/10.1167/iovs.10-5892
PMid:20881305
|
|
|
61 Sakamoto K, Inukai M, Mori A, Nakahara T.
Brilliant Blue G protects against photoreceptor injury in a murine
endotoxin-induced uveitis model. Exp Eye Res 2018;177:45-49.
https://doi.org/10.1016/j.exer.2018.07.028
PMid:30063882
|
|
|
62 Fröhlich T, Reiter C, Ibrahim MM, Beutel
J, Hutterer C, Zeitträger I, Bahsi H, Leidenberger M, Friedrich O, Kappes B,
Efferth T, Marschall M, Tsogoeva SB. Synthesis of novel hybrids of
quinazoline and artemisinin with high activities against Plasmodium
falciparum, human cytomegalovirus, and leukemia cells. ACS Omega
2017;2(6):2422-2431.
https://doi.org/10.1021/acsomega.7b00310
PMid:30023664 PMCid:PMC6044832
|
|
|
63 Breuer E, Efferth T. Treatment of
iron-loaded veterinary sarcoma by Artemisia annua. Nat Prod Bioprospect
2014;4(2):113-118.
https://doi.org/10.1007/s13659-014-0013-7
PMid:24859473 PMCid:PMC4004853
|
|
|
64 Li X, Ba Q, Liu Y, Yue Q, Chen P, Li J,
Zhang H, Ying H, Ding Q, Song H, Liu H, Zhang R, Wang H. Dihydroartemisinin
selectively inhibits PDGFRα-positive ovarian cancer growth and metastasis
through inducing degradation of PDGFRα protein. Cell Discov 2017;3:17042.
https://doi.org/10.1038/celldisc.2017.42
PMid:29387451 PMCid:PMC5787695
|
|
|
65 Kumari K, Keshari S, Sengupta D, Sabat SC,
Mishra SK. Transcriptome analysis of genes associated with breast cancer cell
motility in response to Artemisinin treatment. BMC Cancer 2017;17(1):858.
https://doi.org/10.1186/s12885-017-3863-7
PMid:29246124 PMCid:PMC5732364
|
|
|
66 Zhang T, Hu Y, Wang T, Cai P.
Dihydroartemisinin inhibits the viability of cervical cancer cells by
upregulating caveolin 1 and mitochondrial carrier homolog 2: Involvement of
p53 activation and NAD(P)H: quinone oxidoreductase 1 downregulation. Int J
Mol Med 2017;40(1):21-30.
https://doi.org/10.3892/ijmm.2017.2980
PMid:28498397 PMCid:PMC5466377
|
|
|
67 Kogachi K, Kim JW, Green S, Jubran R,
Berry JL. Lurking below: massive choroidal invasion under a calcified tumor
after attempted conservative therapy for retinoblastoma. Ophthalmic Genet
2018;39(5): 653-657.
https://doi.org/10.1080/13816810.2018.1513535
PMid:30142285 PMCid:PMC6613565
|
|
|
68 Zhao F, Wang H, Kunda P, Chen X, Liu QL,
Liu T. Artesunate exerts specific cytotoxicity in retinoblastoma cells via
CD71. Oncol Rep 2013;30(3):1473-1482.
https://doi.org/10.3892/or.2013.2574
PMid:23818062
|
|
|
69 Arroba AI, Campos-Caro A,
Aguilar-Diosdado M, Valverde ÁM. IGF-1, inflammation and retinal
degeneration: a close network. Front Aging Neurosci 2018;10:203.
https://doi.org/10.3389/fnagi.2018.00203
PMid:30026694 PMCid:PMC6041402
|
|
|
70 Zeng Z, Xu J, Zheng W. Artemisinin protects
PC12 cells against β-amyloid-induced apoptosis through activation of the
ERK1/2 signaling pathway. Redox Biol 2017;12:625-633.
https://doi.org/10.1016/j.redox.2017.04.003
PMid:28391183 PMCid:PMC5385605
|
|
|
71 Chong CM, Zheng W. Artemisinin protects
human retinal pigment epithelial cells from hydrogen peroxide-induced
oxidative damage through activation of ERK/CREB signaling. Redox Biol
2016;9:50-56.
https://doi.org/10.1016/j.redox.2016.06.002
PMid:27372058 PMCid:PMC4939375
|
|
|
72 Yan F, Wang H, Gao Y, Xu J, Zheng W.
Artemisinin protects retinal neuronal cells against oxidative stress and restores
rat retinal physiological function from light exposed damage. ACS Chem
Neurosci 2017;8(8):1713-1723.
https://doi.org/10.1021/acschemneuro.7b00021
PMid:28447781
|
|
|
73 Mesquita J, Castro-de-Sousa JP,
Vaz-Pereira S, Neves A, Passarinha LA, Tomaz CT. Evaluation of the growth
factors VEGF-a and VEGF-B in the vitreous and serum of patients with macular
and retinal vascular diseases. Growth Factors 2018;36(1-2):48-57.
https://doi.org/10.1080/08977194.2018.1477140
PMid:29969324
|
|
|
74 Lekha T, Prasad HN, Sarwate RN, Patel M,
Karthikeyan S. Intravitreal bevacizumab for choroidal neovascularization associated
with angioid streaks: long-term results. Middle East Afr J Ophthalmol
2017;24(3):136-142.
https://doi.org/10.4103/meajo.MEAJO_17_17
PMid:29279654 PMCid:PMC5698988
|
|
|
75 Moreno M, Pow PY, Tabitha TST, Nirmal S,
Larsson A, Radhakrishnan K, Nirmal J, Quah ST, Geifman Shochat S, Agrawal R,
Venkatraman S. Modulating release of ranibizumab and aflibercept from
thiolated chitosan-based hydrogels for potential treatment of ocular
neovascularization. Expert Opin Drug Deliv 2017;14(8):913-925.
https://doi.org/10.1080/17425247.2017.1343297
PMid:28643528
|
|
|
76 El Alaoui-Lasmaili K, Faivre B. Antiangiogenic
therapy: markers of response, "normalization" and resistance. Crit
Rev Oncol Hematol 2018;128:118-129.
https://doi.org/10.1016/j.critrevonc.2018.06.001
PMid:29958627
|
|
|
77 Borkar DS, Obeid A, Su DC, Storey PP,
Gao X, Regillo CD, Kaiser RS, Garg SJ, Hsu J; Wills Post Injection
Endophthalmitis (PIE) Study Group. Endophthalmitis rates after bilateral
same-day intravitreal anti-vascular endothelial growth factor injections. Am
J Ophthalmol 2018;194:1-6.
https://doi.org/10.1016/j.ajo.2018.06.022
PMid:29981738
|
|
|
78 Kumar M, Dhatwalia SK, Dhawan DK. Role
of angiogenic factors of herbal origin in regulation of molecular pathways
that control tumor angiogenesis. Tumour Biol 2016;37(11):14341-14354.
https://doi.org/10.1007/s13277-016-5330-5
PMid:27614685
|
|
|
79 Verma S, Das P, Kumar VL.
Chemoprevention by artesunate in a preclinical model of colorectal cancer
involves down regulation of β-catenin, suppression of angiogenesis, cellular
proliferation and induction of apoptosis. Chem Biol Interact 2017;278:84-91.
https://doi.org/10.1016/j.cbi.2017.10.011
PMid:29031619
|
|
|
80 Zhou HJ, Wang WQ, Wu GD, Lee J, Li A.
Artesunate inhibits angiogenesis and downregulates vascular endothelial growth
factor expression in chronic myeloid leukemia K562 cells. Vascul Pharmacol
2007;47(2-3):131-138.
https://doi.org/10.1016/j.vph.2007.05.002
PMid:17581794
|
|
|
81 Chen HH, Zhou HJ, Wu GD, Lou XE.
Inhibitory effects of artesunate on angiogenesis and on expressions of
vascular endothelial growth factor and VEGF receptor KDR/flk-1. Pharmacology
2004;71(1):1-9.
https://doi.org/10.1159/000076256
PMid:15051917
|
|
|
82 Chen HH, Zhou HJ, Wang WQ, Wu GD.
Antimalarial dihydroartemisinin also inhibits angiogenesis. Cancer Chemother
Pharmacol 2004;53(5): 423-432.
https://doi.org/10.1007/s00280-003-0751-4
PMid:15132130
|
|
|
83 Cheng R, Li C, Li C, Wei L, Li L, Zhang
Y, Yao Y, Gu X, Cai W, Yang Z, Ma J, Yang X, Gao G. The artemisinin
derivative artesunate inhibits corneal neovascularization by inducing
ROS-dependent apoptosis in vascular en dothelial cells. Invest Ophthalmol Vis
Sci 2013;54(5): 3400-3409.
https://doi.org/10.1167/iovs.12-11068
PMid:23611999 PMCid:PMC5963000
|
|
|
84 Zong Y, Yuan Y, Qian X, Huang Z, Yang W,
Lin L, Zheng Q, Li Y, He H, Gao Q. Small molecular-sized artesunate
attenuates ocular neovascularization via VEGFR2, PKCα, and PDGFR targets. Sci
Rep 2016;6:30843.
https://doi.org/10.1038/srep30843
PMid:27480521 PMCid:PMC4969591
|
|