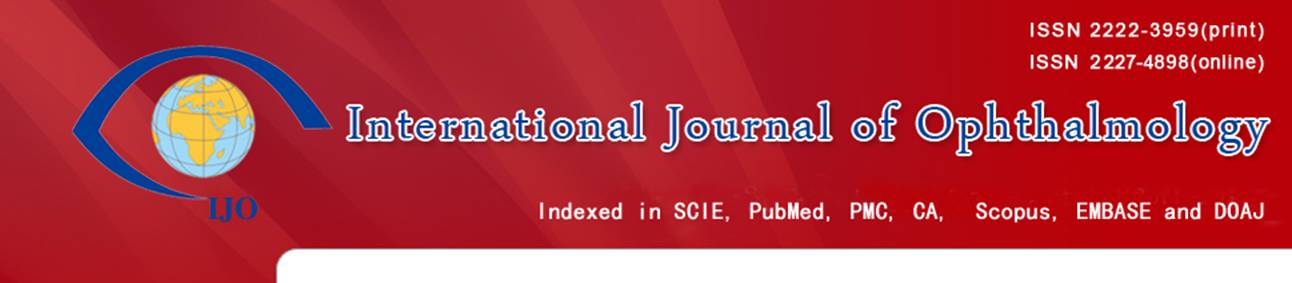
·Review Article·
LncRNAs
in ocular neovascularizations
Yacouba Cissé, Lang Bai, Min-Ting Chen
Department of Ophthalmology, Nanfang
Hospital, Southern Medical University, Guangzhou 510515, Guangdong Province,
China
Correspondence to: Lang Bai. Department of
Ophthalmology, Nanfang Hospital, Southern Medical University, Number 1838
Guangzhou North Avenue, Guangzhou 510515, Guangdong Province, China.
bailangsfy@126.com
Received: 2019-03-01
Accepted: 2019-05-29
Abstract
The prevalence of eye diseases
worldwide is dramatically increasing and represents a major concern in
underdeveloped and developed regions. Ocular diseases, previously associated
with a higher depression risk, also impose a substantial economic burden on
affected families, thus early detection and/or accurate treatment in order to
avoid and prevent blindness should be emphasized. Ocular neovascularization
(NV), the leading cause of blindness in a variety of eye diseases, is a
pathologic process characterized by the formation, proliferation and
infiltration of anomalous, tiny and leaky fragile blood vessels within the eye.
Genetics have been suspected to play an important role in the occurrence of eye
diseases, with the detection of a numbers of specific gene mutations. Long
non-coding RNA (lncRNAs) are novel class of regulatory molecules previously
associated with various biological processes and diseases, however the nature
of the relation and pathways by which they might contribute to the development
of corneal, choroidal and retinal NV have not yet been completely elucidated.
In this review, we focus on the regulation and characteristics of lncRNAs,
summarize results from ocular NV-related studies and discuss the implication of
lncRNAs in ocular NV development.
KEYWORDS: glaucoma; genetics; long non-coding RNA;
neovascularization
DOI:10.18240/ijo.2019.12.19
Citation: Cissé
Y, Bai L, Chen MT. LncRNAs in ocular neovascularizations. Int J Ophthalmol
2019;12(12):1959-1965
INTRODUCTION
The prevalence of eye diseases
worldwide is dramatically increasing and represents a major concern in
underdeveloped and developed regions, especially sight threatening diseases.
Ocular diseases, previously associated with an higher depression risk, also impose
a substantial economic burden on affected families, thus the importance of
early detection and/or accurate treatment in order to avoid and prevent
blindness should be emphasized[1]. Ocular
neovascularization (NV), the leading cause of blindness in a variety of eye
diseases, is a pathologic process characterized by the formation, proliferation
and infiltration of anomalous, tiny and leaky fragile blood vessels within the
eye[2]. Occurrence of eye diseases have been
assigned to a number of specific gene mutations such as EIF1AX, SF3B1,
PLCB4, GNA11, BAP1 and GNAQ for uveal melanoma[3-5], and RB1 for retinoblastoma[6], however, not all diseases exhibit a
mendelian mode of inheritances. The non-coding part of the human genome has
recently been recognized to possess a crucial functional importance in
physiology and normal development. And this discovery has attracted attention
on its potential to contribute to diverse disease etiology[7].
Long non-coding RNA (lncRNAs) are transcripts possessing a size within 200 to
100 000 nucleotides, structurally resembling mRNA and presenting little to no
protein-coding potential and can be classified into several types according to
their genonic locations. Although most lncRNAs are located in the nucleus[8], however nearly 15% can be found present in the
cytoplasm[9]. LncRNAs can be classified as sense
or antisense, the former comprising of those that overlap with proteing-coding
genes. If the promoter and transcript are situated in proximity, the lncRNA is
then said to be bidirectional[10-11].
Many studies reported lncRNas
participation in numerous biological processes, including stem cell
maintenance, and cellular phenotype differentiation[12].
Transcriptinal regulation may be influenced by LncRNAs via several modes
such as decoy, signal, guide and scaffold[13].
They might also as signals in response to multiple stimuli, participate in
recruiting corresponding complexes in order to directly or indirectly silence
or activate the expression of a gene[14]. In
addition, some lncRNAs may influence the expression of genes through
post-transcriptional events, and also participate in the modification process
post translation[15]. Nonetheless, lncRNAs have
been previously associated with neurodegenerative diseases[16],
multiple tumors and cancers[17-18],
and common ocular diseases such glaucoma[19], and
diabetic retinopathy[20], among others, however
the nature of the relation and pathways by which they might contribute to the
development of corneal, choroidal and retinal NV have not yet been completely
elucidated.
In this review, we review
pathophysiology and risk factors for developing ocular NVs focus on the
regulation and characteristics of lncRNAs, summarize results from ocular
NV-related studies and discuss the implication of lncRNAs in ocular NV
development
LNCRNAS IN OCULAR NEOVASCULARIZATIONS
Corneal Neovascularization
Definition Corneal diseases are considered one of
the main causes of irreversible blindness, and corneal neovascularization (CN)
can be observed in the majority of affected cases. Further investigations have
shown that angiogenesis negatively impacts the prognosis for individuals
undergoing keratoplasty procedures[2].
Normal corneal transparency is an
essential factor in providing appropriate anterior refractive surface. Corneal
transparency and optimal vision require an important aspect of corneal
pathophysiology which is the avascularity of the corneal stroma. Decrease in
corneal transparency associated with the development of NV. The presence of
vascularization, is mostly linked to pathologic conditions. And may cause
visual acuity deterioration[21]. Characterized
by the development and invasion of neovessels-from the limbus to the cornea, CN
mainly results from oxygen deprivation provoked by disequilibrium between
corneal transparency preserving factors and lead to proliferation and migration
of vascular endothelial cells into the corneal stroma. The newly formed vessels
may then cause inflammation, oedema, protein and lipid deposition, therefore
posing a considerable threat to both visual acuity and corneal transparency
and, contribute to corneal grafts rejection[22-24]. It is currently estimated that approximately
1.4 million new cases of CN are registered every year, 12% of whom eventually
leading to visual loss[25].
Pathogenesis The
upregulation of angiogenic cytokines mediates the in-growth of new blood
vessels. Cornea’s basement membrane and extracellular matrix are degraded by
the metalloproteinase enzyme, while the vascular epithelial cells invasion into
corneal’s stromal layer is facilitated by proteolytic enzymes. Inflammatory
cells produce angiogenic factors such as VEGF and fibroblast growth factors
during inflammation process. With the former regulating the production of
matrix metalloproteinases by endothelial cells, thus paving the way for the
formation of new blood vessel[26].
Risk factors CN can be
caused by congenital diseases, autoimmune conditions, inflammatory disorders,
infections, hypoxia, chemical burn, limbal stem cell deficiency, corneal graft
rejection, and traumas. CN within the cornea usually results from ulcers,
trachoma, different types of keratitis and conjunctivitis. Superficial
presentations of CN usually results from hypoxia caused by contact lenses,
whereas chronic inflammatory and anterior segment ocular diseases are
considered main causes for deep presentations of CN[27].
LncRNAs in corneal neovascularization
LncRNA NR_033585 and lincRNA chr8:129102060–129109035 A recent study[28] tried to highlight the
possible role played by lncRNAs in the development of CN, and consisted in
using alkaline solution to stimulate CN development in C57BL/6 mice eyes and
lncRNA expression profiling in order to compare expression differences in lnRNA
among normal and vascularized corneas. The observation of approximately 154
differentialy expressed lncRNAs then prompted researchers to randomly selected
a number of 6 lncRNAs (3 up-regulated and 3 down-regulated) and proceed to a
comparison between their expression patterns and antiangiogenic factors and
proangiogenic factors[29]. The result showed
similar expression pattern between proangiogenic factors, such as Ang-2, MMP-9
and VEGF, and up-regulated lncRNAs (lncRNA NR_033585), whereas down-regulated
lncRNAs (lincRNA chr8:129102060-129109035) did exhibit expression patterns that
were similar to antiangiogenic factors such as platelet derived growth factor
(PDGF)[30].
Further investigations conducted
with the aim to explore the possible correlation existing between abberant
lncRNAs expression and CN development suggest that lncRNA NR_033585 may
potentially act as an proangiogenic factor, whereas the reverse strand of
lincRNA: chr8:129102060-129109035 might play an antiangiogenic role during the
development process of CN[31].
Choroidal Neovascularization
Definition Choroid is located between the retina
and the sclera. Its main function consists of supplying nourishment and
moistening the volume and temperature of the ocular globe. Despite the fact
that the choroidal circulation is a relatively low oxygen content, it still
accounts for nearly 85% of the total blood flow in the eye[32].
choroidal neovascularization (CNV),
a pathologic condition whose main characteristics are abnormal growth of new
blood vessels in the choroid, and if left untreated, it can cause a rapid
deterioration in central vision and color recognition especially when the
subfoveal region is being affected[33]. CNV is
considered a main cause of central visual loss in both adults[34] and children[35-36]; despite possessing a lower blindness prevalence,
however children, because of a higher disability-adjusted life years (DALY)[37] have been shown to face greater challenges not only
in education but also in emotional development[38-39].
New blood vessels, formed from the
choroid, penetrate into the subretinal pigment epithelial and subretinal space
through the Bruch membrane. Bleeding and leaking from these newly formed
vessels can lead to hemorrhage and to exudative retinal detachment[40], and may cause destruction of photoreceptors and
vision loss (blindness). Although CNV is considered to be a multifactorial
lesion and its development is known to be induced by numerous stimuli, the
involvement of macrophages and VEGF and alterations in Bruch’s membrane, take
the main role for the development of this disease[41].
Pathogenesis Vascularization in the choroid develop
when newly formed blood vessels start to grow into the sub-retinal space
through a break in the Bruch membrane. The presence of abnormaly elevated VEGF
levels, and its counteraction by retinal epithelia produced protein, also
called pigment epithelium derived factor (PEDF) are the main inducer of new
blood vessels growth. Maintening the balance between VEGF and PEDF is thought
to be one of the main determining factor in the development or progression of
CNV[42-43].
Risk factors CNV can be observed in various ocular
diseases including adult macular degeneration (AMD)[44],
pathologic myopia (PM)[45] and ocular
histoplasmosis syndrome (OHS)[46]. However,
choroid’s primary functions can be affected by any condition capable of
disrupting the Bruch’s membrane, thus increasing the risk of developing CNV.
The incidence and progression of AMD are related to age and genetic factors[47]. The activity of lysosomes for external segments
degradation of photoreceptors decreases proportionally with age, ultimately
leading to an lipofuscin accumulation, thus affecting normal function of
retinal pigment epithelium (RPE)[48].
Furthermore, soft drusen represent another important risk factor for CNV
development[49].
Several other risk factors have been
previously been reported, including age[50],
smoking[51], hyperglycemia[52],
dietary intake of omega-3 fatty acids[53] and vegetables
and fruit with antioxidants including lutein[54]
and zeaxanthin[55], as well as, hereditary or
traumatic or inflammatory disorders[56].
LncRNAs in choroidal neovascularization
Vax2os1 and Vax2os2 A recent research tried to investigate the possible changes in VEGF and
lncRNAs expression via the exposition of mice to hyperoxic condition for
a period of 5d in order to induce
ocular NV; whereas age-matched control groups were kept in room air. The result
showed disappearance of existing capillaries after exposure to 75%±2% oxygen,
even though peripheral retina remained vascularized. Microarray data
demonstrated that lncRNAs expression level were different among case and control
group[57].
Furthermore, aqueous humor samples
were collected from patients affected with exudative AMD (case, n=10)
and those with cataract surgery (control, n=10) and later submitted to
microarray analysis and revealed a significant up-regulation in Vax2os1,Vax2os2
and VEGF expression, among patients presenting ocular NV, thus suggesting their
possible importance as potential biomarkers[58].
Vax2os1 and Vax2os2, are antisense
transcripts of the Vax2 gene, highly expressed in the choroid and retinal
vasculature. A strong RNA-protein interactions existing not only between
Vax2os1 and C1D, but also between Vax20s2 and PATL2, might play a undeniably
important part in CNV pathogeneisis because C1D and PATL2 serve as important
regulators of chromatin structure stability[59-60]. Increased information about these two lncRNAs will
facilitate a greater understanding of CNV pathogenesis. Provided that each lncRNA
regulates specific facets of protein activity, a more refined and less toxic
drug targeting a lncRNA may be employed for CNV treatment.
Retinal Neovascularization
Definition Retinal NV refers to the formation of
neovessels originating from and contiguous with the pre-existing retinal
vascular bed and generally occurs in ischemic retinopathies in which the damage
to retinal vessels results in retinal ischemia.
Pathogenesis The newly
formed blood vessels are located either within or near the retina, in portions
where blood vessels are not usually present. Such new vessels are actually
generated from the blood vessels in the retina and the NV may remain within the
plane of the inner retina, grow inwards toward the vitreal surface and escape
from the internal limiting membrane, or extend downward to the inner nuclear
layer and go as far as the RPE.
Risk factors The most prevalent is risk factors for
retinal NV are: diabetic retinopathy, retinal vein occlusions[61]. Other risk factors include Behcet’s disease[62], sickle cell hemoglobinopathy[63],
sarcoidosis[64], Eales disease[65],
and systemic lupus erythematosus[66].
LncRnas in retinal neovascularizations Myocardial infarction-associated transcript (MIAT) has
been previously associated with myocardial infarction ,is reportedly highly
expressed in retinal precursor cells[67].
Yan et al[68] tried
highlighted its involvement in microvascular dysfunction caused by diabetes
mellitus through quantitative polymerase chain reaction (PCR) to evaluated its
expression in both diabetic retinas and endothelial cells placed in high
glucose medium. Their research not only revealed an up-regulation of MIAT level
post high-glucose or oxidative stress treatment and also a proliferation and
migration of endothelial cell ultimately causing microvascular dysfunction this
up-regulation contributes to endothelial cell proliferation and migration, thus
leading to microvascular dysfunction. Further investigation on therapeutic
effects of prompted them to perform an MIAT down-regulation which led to
inhibition of endothelial inflammatory responses. This might be due to the fact
that MIAT might act as competing endogenous RNA in VEGF regulation and thus
participate in the development of retinal NV. A recent hypothesis also states
that by competing specifically for shared miRNAs, RNA transcripts sharing
miRNA-binding might be able to communicate and regulate each other[69]. Which could explain how MIAT by binding to the same
site as miR-150-5p, might alleviate repression effect of miR-150-5p and cause
up-regulation of its target gene VEGF. Additionally, inhibition of upregulation
of both TNF-α and ICAM-1 can be provoked by MIAT knockdown,thus reducing
inflammaton and vascular leakeage[70].
MALAT1 is a long non-coding RNA highly
expressed in the nucleus, and located on chromosome 11q13[71]
in human, and 19qA[72] in mouse. It is one of the
most studied lncRNAs and has been identified in a wide range of tumors,
including lung cancer, liver cancer, renal cell carcinoma, bladder cancer, and
osteosarcoma[73], and microvascular dysfunction
caused by diabetes[74]. The expression level of MALAT1
has been shown to be similar to protein-coding genes such as β-actin,
glyceraldehyde 3-phosphate dehydrogenase (GAPDH)[75],
and this findng is the main catalyst for the conduction of large amount of
researches in order to provide underlyning insights of its functions.
Gene epression can be regulated by MALAT1,
which has also been showed to play a positive role in tumor cell proliferation,
invasion, migration and apoptosis[76]. Recent
studies not only showed that the cerebellum, hippocampus and brain stem cells
of alcoholics, and tumors affected patents harbor up-regulated level of MALAT1,
which hints at its possible role in neurodegeneration[77],
but also revealed that hypoxia, hyperglycemia and oxygen deprivation are the
main causes of its up-regulation[78].
Furthermore, a genetic MALAT1
deletion in mice resulted in a reduction of retinal vascular growth and the
presentation of an endothelial proliferation. Furthermore, both cultured mouse
primary skeletal muscle microvascular endothelial cells followed by 24h
reperfusion and mouse gastrocnemius muscle that underwent hindlimb ischemia
followed by 28d of reperfusion were shown to harbor increased level of both MALAT1
and VEGFR2. MALAT1 silencing through a locked nucleic acid
(LNA)-GapmeRs method, leads to a reduction in tube formation, cell
proliferation and migration, suggesting an important role played by MALAT1 in angiogenesis through direct regulation of VEGFR2 and
that genetic MALAT1 deficiency could provoke an angiogenesis
reduction and perfusion[79].
Yan et al[80] were
among the first to perform lncRNA expression profiling of mouse retinas with
the aim to identify the possible lncRNAs implication in early diabetic
retinopathy, followed by the conduction of a real-time PCR for the detection
and comparison of expression pattern between clinical samples and RF/6A cell model of hyperglycemia. The
results showed aberrant expression in approximately 303 lncRNAs (comprising of
214 down-regulated and 89 up-regulated), in early diabetic retinopathy.
Additionally, MALAT1, was not only found to be up-regulated in the mouse model
of hpyperglycema, but also in both the aqueous humor and fibrovascular
membranes of diabetes-affected patients. LncRNAs by participating in the
modulation of numerous pathogenic pathways contribute to the pathogenesis of
diabetic retinopathy. MALAT1 could possibly serve as a therapeutic target for
the prognosis, diagnosis and treatment of diabetic retinopathy[22].
Liu et al[81] emphasized
the role played by MALAT1 in
retinal vasculature remodeling by demonstrating its upregulated expression in
both STZ-induced diabetic rats and db/db mice retinas. Furthermore, the
crosstalk between MALAT1 and p38 MAPK signaling pathway being involved in the
regulation of endothelial cell function, an MALAT1 knockdown could alleviate in
vivo microvascular dysfunction and through affecting phosphorylated p38
MAPKs levels provokes inhibition of tube formation, endothelial cell
proliferation, ultimately ameliorating prognosis of diabetic retinopathy[79].
Michalik et al[82]
focused their researches on expression characterization of MALAT1 in human endothelial cells). The study
showed an upregulation of MALAT1 level post hypoxia induction, and also
confirmed that the endothelial cells proliferation and neonatal retinal
vascularization could be respectively inhibited and reduced after an MALAT1
genetic ablation, whereas a pharmacological MALAT1 inhibition causes the
reduction of blood flow recovery and capillary density.
CONCLUSION
Ocular NV, the leading cause of
blindness in a variety of eye diseases, is a pathologic process characterized
by the formation of anomalous blood vessels within the eye. LncRNAs are novel
class of regulatory molecules previously associated with various biological
processes and c diseases, however the nature of the relation and pathways by
which they might contribute to the development of corneal, choroidal and
retinal NV have not yet been completely elucidated.
In summary, we discussed potential
implications of lncRNAs such as Vax2os1, Vax2os2, lncRNA NR_033585, lincRNA chr8:129102060-129109035,
MIAT, and MALAT1 in the
development of ocular NVs. Nevertheless, more in depth research are needed to
identify large number of ocular NV-associated lncRNAs and elucidate their
involvement in the development of this condition. We stress that this article
creates a paradigm for future studies of lncRNAs in the prevention, diagnosis
and might provide therapeutic targets for treatments, and help avoid vison loss
and vsual impairment caused by ocular NV.
ACKNOWLEDGEMENTS
Authors’ contributions: In conception, design (Cissé Y),
literature search and writing of the manuscript (Cissé Y); supervision (Bai L),
critical reading (Bai L, Chen MT), approval of the final proofs of the article
(Cissé Y, Bai L, Chen MT).
Foundations: Supported by the National Natural
Science Foundation of China (No.81170887); the Natural Science Foundation of
Guangdong Province (No.2017A030313602);
the Horizontal Topic Matching Funds of Nanfang Hospital, Southern Medical
University (No.G201202).
Conflicts of Interest: Cissé Y, None; Bai L, None; Chen
MT, None.
REFERENCES
1 McCusker S, Koola MM. Association of ophthalmologic
disorders and depression in the elderly. Prim Care Companion CNS Disord
2015;17(4).
https://doi.org/10.4088/PCC.14r01731
PMid:26693037 PMCid:PMC4664565
|
|
2 Zhang Y, Cai S, Jia Y, Qi C, Sun J, Zhang
H, Wang F, Cao Y, Li X. Decoding noncoding RNAs: role of microRNAs and long
noncoding RNAs in ocular neovascularization. Theranostics
2017;7(12):3155-3167.
https://doi.org/10.7150/thno.19646
PMid:28839470 PMCid:PMC5566112
|
|
|
3 Helgadottir, H, Höiom V. The genetics of
uveal melanoma: current insights. Appl Clin Genet 2016;9:147-155.
https://doi.org/10.2147/TACG.S69210
PMid:27660484 PMCid:PMC5019476
|
|
|
4 Schneider B, Riedel K, Zhivov A, Huehns
M, Zettl H, Guthoff RF, Jünemann A, Erbersdobler A, Zimpfer A. Frequent and yet
unreported GNAQ and GNA11 mutations are found in uveal melanomas. Pathol
Oncol Res 2019;25(4):1319-1325.
https://doi.org/10.1007/s12253-017-0371-7
PMid:29209985
|
|
|
5 Ewens KG, Lalonde E, Richards-Yutz J,
Shields CL, Ganguly A. Comparison of germline versus somatic BAP1 mutations
for risk of metastasis in uveal melanoma. BMC Cancer 2018;18(1):1172.
https://doi.org/10.1186/s12885-018-5079-x
PMid:30477459 PMCid:PMC6260582
|
|
|
6 Kalsoom S, Wasim M, Afzal S, Shahzad MS,
Ramzan S, Awan AR, Anjum AA, Ramzan K. Alterations in the RB1 gene in Pakistani
patients with retinoblastoma using direct sequencing analysis. Mol Vis
2015;21:1085-1092.
|
|
|
7 Beermann J, Piccoli MT, Viereck J, Thum
T. Non-coding RNAs in development and disease: background, mechanisms, and
therapeutic approaches. Physiol Rev 2016;96(4):1297-1325.
https://doi.org/10.1152/physrev.00041.2015
PMid:27535639
|
|
|
8 Zhang K, Shi ZM, Chang YN, Hu ZM, Qi HX, Hong
W. The ways of action of long non-coding RNAs in cytoplasm and nucleus. Gene
2014;547(1):1-9.
https://doi.org/10.1016/j.gene.2014.06.043
PMid:24967943
|
|
|
9 Rashid F, Shah A, Shan G. Long non-coding
RNAs in the cytoplasm. Genom Proteom Bioinform 2016;14(2):73-80.
https://doi.org/10.1016/j.gpb.2016.03.005
PMid:27163185 PMCid:PMC4880952
|
|
|
10 Panzeri I, Rossetti G, Abrignani S,
Pagani M. Long intergenic non-coding rnas: novel drivers of human lymphocyte
differentiation. Front Immunol 2015;6:175.
https://doi.org/10.3389/fimmu.2015.00175
PMid:25926836 PMCid:PMC4397839
|
|
|
11 Grote P, Herrmann BG. Long noncoding
RNAs in organogenesis: making the difference. Trends Genet
2015;31(6):329-335.
https://doi.org/10.1016/j.tig.2015.02.002
PMid:25743487
|
|
|
12 Ramos AD, Andersen RE, Liu SJ,
Nowakowski TJ, Hong SJ, Gertz C, Salinas RD, Zarabi H, Kriegstein AR, Lim DA.
The long noncoding RNA Pnky regulates neuronal differentiation of embryonic and
postnatal neural stem cells. Cell Stem Cell 2015;16(4):439-447.
https://doi.org/10.1016/j.stem.2015.02.007
PMid:25800779 PMCid:PMC4388801
|
|
|
13 Fang Y, Fullwood MJ. Roles, functions,
and mechanisms of long non-coding RNAs in cancer. Genomics Proteomics
Bioinformatics 2016;14(1):42-54.
https://doi.org/10.1016/j.gpb.2015.09.006
PMid:26883671 PMCid:PMC4792843
|
|
|
14 Amicone L, Citarella F, Cicchini C.
Epigenetic regulation in hepatocellular carcinoma requires long noncoding
RNAs. Biomed Res Int 2015;2015:473942.
https://doi.org/10.1155/2015/473942
PMid:25861629 PMCid:PMC4377435
|
|
|
15 Huarte M. The emerging role of lncRNAs
in cancer. Nat Med 2015;21(11):1253-1261.
https://doi.org/10.1038/nm.3981
PMid:26540387
|
|
|
16 Lourenco GF, Janitz M, Huang Y, Halliday
GM. Long noncoding RNAs in TDP-43 and FUS/TLS-related frontotemporal lobar
degeneration (FTLD). Neurobiol Dis 2015;82:445-454.
https://doi.org/10.1016/j.nbd.2015.07.011
PMid:26220395
|
|
|
17 Kumar M, DeVaux RS, Herschkowitz JI.
Molecular and cellular changes in breast cancer and new roles of lncRNAs in breast
cancer initiation and progression. Prog Mol Biol Transl Sci 2016;144:563-586.
https://doi.org/10.1016/bs.pmbts.2016.09.011
PMid:27865468
|
|
|
18 Huang X, Zhi X, Gao Y, Ta N, Jiang H,
Zheng J. LncRNAs in pancreatic cancer. Oncotarget 2016;7(35):57379-57390.
https://doi.org/10.18632/oncotarget.10545
PMid:27429196 PMCid:PMC5302996
|
|
|
19 Cissé Y, Bai L, Meng T. LncRNAs in
genetic basis of glaucoma. BMJ Open Ophthalmol 2018;3(1):e000131.
https://doi.org/10.1136/bmjophth-2017-000131
PMid:29963644 PMCid:PMC6020790
|
|
|
20 Raut SK, Khullar M. The big entity of
new RNA world: long non-coding RNAs in microvascular complications of
diabetes. Front Endocrinol (Lausanne) 2018;9:300.
https://doi.org/10.3389/fendo.2018.00300
PMid:29915562 PMCid:PMC5994400
|
|
|
21 Abdelfattah NS, Amgad M, Zayed AA, Salem
H, Elkhanany AE, Hussein H, Abd El-Baky N. Clinical correlates of common
corneal neovascular diseases: a literature review. thalmol 2015;8(1): 182-193.
|
|
|
22 Usui T, Sugisaki K, Iriyama A, Yokoo S,
Yamagami S, Nagai N, Ishida S, Amano S. Inhibition of corneal
neovascularization by blocking the angiotensin II type 1 receptor. Invest
Ophthalmol Vis Sci 2008;49(10):4370-4376.
https://doi.org/10.1167/iovs.07-0964
PMid:18829859
|
|
|
23 Bachmann B, Taylor RS, Cursiefen C.
Corneal neovascularization as a risk factor for graft failure and rejection
after keratoplasty: an evidence-based meta-analysis. Ophthalmology
2010;117(7):1300-1305.e7.
https://doi.org/10.1016/j.ophtha.2010.01.039
PMid:20605214
|
|
|
24 Inomata T, Mashaghi A, di Zazzo A, Lee SM,
Chiang H, Dana RZ. Kinetics of angiogenic responses in corneal
transplantation. Cornea 2017;36(4):491-496.
https://doi.org/10.1097/ICO.0000000000001127
PMid:28060028 PMCid:PMC5334361
|
|
|
25 Lee P, Wang CC, Adamis AP. Ocular
neovascularization: an epidemiologic review. Surv Ophthalmol
1998;43(3):245-269.
https://doi.org/10.1016/S0039-6257(98)00035-6
|
|
|
26 Liu X, Wang S, Wang X, Liang J, Zhang Y.
Recent drug therapies for corneal neovascularization. Chem Biol Drug Des
2017;90(5):653-664.
https://doi.org/10.1111/cbdd.13018
PMid:28489275
|
|
|
27 Liu S, Romano V, Steger B, Kaye SB,
Hamill KJ, Willoughby CE. Gene-based antiangiogenic applications for corneal
neovascularization. Surv Ophthalmol 2018;63(2):193-213.
https://doi.org/10.1016/j.survophthal.2017.10.006
PMid:29080632
|
|
|
28 Huang J, Li Y, Liu J, Zhang Y, Li X,
Wang L, Yao J, Yan B. Identification of corneal neovascularization-related long
noncoding RNAs through microarray analysis. Cornea 2015;34(5):580-587.
https://doi.org/10.1097/ICO.0000000000000389
PMid:25747163
|
|
|
29 Lu P, Li L, Liu G, Baba T, Ishida Y,
Nosaka M, Kondo T, Zhang X, Mukaida N. Critical role of TNF-α-induced
macrophage VEGF and iNOS production in the experimental corneal
neovascularization. Invest Ophthalmol Vis Sci 2012;53(7):3516-3526.
https://doi.org/10.1167/iovs.10-5548
PMid:22570350
|
|
|
30 Reiner A, Fitzgerald MEC, Del Mar N, Li
C. Neural control of choroidal blood flow. Prog Retin Eye Res 2018;64:96-130.
https://doi.org/10.1016/j.preteyeres.2017.12.001
PMid:29229444 PMCid:PMC5971129
|
|
|
31 Fine SL, Wood WJ, Isernhagen RD,
Singerman LJ, Bressler NM, Folk JC, Kimura AE, Fish GE, Maguire MG, Alexander
J. Laser treatment for subfoveal neovascular membranes in ocular
histoplasmosis syndrome: results of a pilot randomized clinical trial. Arch
Ophthalmol 1993;111(1):19-20.
https://doi.org/10.1001/archopht.1993.01090010021006
PMid:7678731
|
|
|
32 Zhao S, Lan XW, Wu JY, Yue S, Zhang H,
Wu Q, Zhang GS, Liu L. Protocol of global incidence and progression of age-related
macular degeneration: a systematic review. Medicine (Baltimore)
2019;98(10):e14645.
https://doi.org/10.1097/MD.0000000000014645
PMid:30855452 PMCid:PMC6417534
|
|
|
33 Elkhoyaali A, Chatoui S, Bercheq N,
Elouatassi N, Zerrouk R, Elasri F, Reda K, Oubaaz A. Choroidal
neovascularization complicating Best's vitelliform macular dystrophy in a
child. J Fr Ophtalmol 2016;39(1):69-73.
https://doi.org/10.1016/j.jfo.2015.05.008
PMid:26707756
|
|
|
34 Agarwal A, Invernizzi A, Singh RB,
Foulsham W, Aggarwal K, Handa S, Agrawal R, Pavesio C, Gupta V. An update on inflammatory
choroidal neovascularization: epidemiology, multimodal imaging, and
management. J Ophthalmic Inflamm Infect 2018;8(1):13.
https://doi.org/10.1186/s12348-018-0155-6
PMid:30209691 PMCid:PMC6135736
|
|
|
35 López-Corell PM, Pascual-Camps I,
Martínez-Rubio C, Molina-Pallete R, Barranco-González H. Optic nerve head
drusen associated with neovascular membrane in a child: a case report. Arch
De La Sociedad Española De Oftalmol Engl Ed 2018;93(9):463-466.
https://doi.org/10.1016/j.oftale.2018.06.008
|
|
|
36 Goshorn EB, Hoover DL, Eller AW, Friberg
TR, Jarrett WH 2nd, Sorr EM. Subretinal neovascularization in children and
adolescents. J Pediatr Ophthalmol Strabismus 1995;32(3):178-182.
|
|
|
37 Cass D, Sonksen M, McConachie R.
Developmental setback in severe visual impairment. Arch Dis Child
1994;70(3):192-196.
https://doi.org/10.1136/adc.70.3.192
PMid:7510945 PMCid:PMC1029739
|
|
|
38 Dale N, Sonksen P. Developmental
outcome, including setback, in young children with severe visual impairment. Dev
Med Child Neurol 2002;44(9):613-622.
https://doi.org/10.1111/j.1469-8749.2002.tb00846.x
PMid:12227616
|
|
|
39 Khanani AM, Gahn GM, Koci MM, Dang JM, Brown
SM, Hill LF. Five-year outcomes of intravitreal drug therapy for neovascular
age-related macular degeneration in eyes with baseline vision 20/60 or
better. Clin Ophthalmol 2019;13:347-351.
https://doi.org/10.2147/OPTH.S191170
PMid:30858684 PMCid:PMC6387604
|
|
|
40 Campochiaro PA, Aiello LP, Rosenfeld PJ.
Anti-vascular endothelial growth factor agents in the treatment of retinal
disease: from bench to bedside. Ophthalmology 2016;123(10S):S78-S88.
https://doi.org/10.1016/j.ophtha.2016.04.056
PMid:27664289
|
|
|
41 Yu YJ, Mo B, Liu L, Yue YK, Yue CL, Liu W.
Inhibition of choroidal neovascularization by lentivirus-mediated PEDF gene
transfer in rats. thalmol 2016;9(8):1112-1120.
|
|
|
42 Fan X, Gao N, Li J, Lei J, Kang Q.
Effects of VEGF levels on anti-VEGF therapy for patients with idiopathic
choroidal neovascularization. Mol Cell Biochem 2018;441(1-2):173-179.
https://doi.org/10.1007/s11010-017-3183-x
PMid:28884436
|
|
|
43 Ferris FL, Fine SL, Hyman L. Age-related
macular degeneration and blindness due to neovascular maculopathy. Arch
Ophthalmol 1984;102(11):1640-1642.
https://doi.org/10.1001/archopht.1984.01040031330019
PMid:6208888
|
|
|
44 Schargus M, Pauleikhoff D, Haeusser-Fruh
G, Maier MM. Choroidal neovascularisation in pathological myopia:
epidemiological data from a health services research study conducted in
Germany. Klin Monbl Augenheilkd 2013;230(7):707-714.
https://doi.org/10.1055/s-0032-1328254
PMid:23670525
|
|
|
45 Diaz RI, Sigler EJ, Rafieetary MR,
Calzada JI. Ocular histoplasmosis syndrome. Surv Ophthalmol
2015;60(4):279-295.
https://doi.org/10.1016/j.survophthal.2015.02.005
PMid:25841248
|
|
|
46 SanGiovanni JP, Chew EY. Clinical
applications of age-related macular degeneration genetics. Cold Spring Harb
Perspect Med 2014;4(10):a017228.
https://doi.org/10.1101/cshperspect.a017228
PMid:25125423 PMCid:PMC4200209
|
|
|
47 Terman A, Brunk UT. Lipofuscin:
mechanisms of formation and increase with age. APMIS 1998;106(2):265-276.
https://doi.org/10.1111/j.1699-0463.1998.tb01346.x
PMid:9531959
|
|
|
48 Lee JH, Lee T, Lee SC, Lee CS. Disappearance
of soft drusen and subsequent development of choroidal neovascularization
following macular hole surgery: a case report. BMC Ophthalmol 2015;15:43.
https://doi.org/10.1186/s12886-015-0029-8
PMid:25928705 PMCid:PMC4424516
|
|
|
49 Liu B, Zhang X, Peng Y, Mi L, Wen F.
Etiologies and characteristics of choroidal neovascularization in young
Chinese patients. Ophthalmologica 2019;241(2):73-80.
https://doi.org/10.1159/000492133
PMid:30153680
|
|
|
50 Chheda LV, Ferketich AK, Carroll CP,
Moyer PD, Kurz DE, Kurz PA. Smoking as a risk factor for choroidal neovascularization
secondary to presumed ocular histoplasmosis syndrome. Ophthalmology
2012;119(2):333-338.
https://doi.org/10.1016/j.ophtha.2011.08.003
PMid:22035582
|
|
|
51 Li X, Cai Y, Wang YS, Shi YY, Hou W, Xu
CS, Wang HY, Ye Z, Yao LB, Zhang J. Hyperglycaemia exacerbates choroidal
neovascularisation in mice via the oxidative stress-induced activation of
STAT3 signalling in RPE cells. PLoS One 2012;7(10):e47600.
https://doi.org/10.1371/journal.pone.0047600
PMid:23094067 PMCid:PMC3477109
|
|
|
52 Yanai R, Chen S, Uchi SH, Nanri T, Connor
KM, Kimura K. Attenuation of choroidal neovascularization by dietary intake
of ω-3 long-chain polyunsaturated fatty acids and lutein in mice. PLoS One
2018;13(4):e0196037.
https://doi.org/10.1371/journal.pone.0196037
PMid:29694386 PMCid:PMC5919067
|
|
|
53 Izumi-Nagai K, Nagai N, Ohgami K,
Satofuka S, Ozawa Y, Tsubota K, Umezawa K, Ohno S, Oike Y, Ishida S. Macular
pigment lutein is antiinflammatory in preventing choroidal neovascularization.
Arterioscler Thromb Vasc Biol 2007;27(12):2555-2562.
https://doi.org/10.1161/ATVBAHA.107.151431
PMid:17932319
|
|
|
54 Tan JS, Wang JJ, Flood V, Rochtchina E, Smith
W, Mitchell P. Dietary antioxidants and the long-term incidence of
age-related macular degeneration: the blue mountains eye study. Ophthalmology
2008;115(2):334-341.
https://doi.org/10.1016/j.ophtha.2007.03.083
PMid:17664009
|
|
|
55 Wang WQ, Gawlik K, Lopez J, Wen C, Zhu
J, Wu F, Shi W, Scheibler S, Cai HM, Vairavan R, Shi A, Haw W, Ferreyra H,
Zhang M, Chang S, Zhang K. Genetic and environmental factors strongly
influence risk, severity and progression of age-related macular degeneration.
Signal Transduct Target Ther 2016;1:16016.
https://doi.org/10.1038/sigtrans.2016.16
PMid:29263899 PMCid:PMC5661646
|
|
|
56 Xu XD, Li KR, Li XM, Yao J, Qin J, Yan
B. Long non-coding RNAs: new players in ocular neovascularization. Mol Biol
Rep 2014;41(7): 4493-4505.
https://doi.org/10.1007/s11033-014-3320-5
PMid:24623407
|
|
|
57 Meola N, Pizzo M, Alfano G, Surace EM,
Banfi S. The long noncoding RNA Vax2os1 controls the cell cycle progression
of photoreceptor progenitors in the mouse retina. RNA 2012;18(1):111-123.
https://doi.org/10.1261/rna.029454.111
PMid:22128341 PMCid:PMC3261733
|
|
|
58 Scheller N, Resa-Infante P, de la Luna
S, Galao RP, Albrecht M, Kaestner L, Lipp P, Lengauer T, Meyerhans A, Díez J.
Identification of PatL1, a human homolog to yeast P body component Pat1.
Biochim Biophys Acta 2007;1773(12):1786-1792.
https://doi.org/10.1016/j.bbamcr.2007.08.009
PMid:17936923
|
|
|
59 Tian Y, Simanshu DK, Ascano M,
Diaz-Avalos R, Park AY, Juranek SA, Rice WJ, Yin Q, Robinson CV, Tuschl T,
Patel DJ. Multimeric assembly and biochemical characterization of the Trax-translin
endonuclease complex. Nat Struct Mol Biol 2011;18(6):658-664.
https://doi.org/10.1038/nsmb.2069
PMid:21552261 PMCid:PMC3109869
|
|
|
60 Pierru A, Girmens JF, Héron E, Paques M.
Retinal vein occlusions. J Fr Ophtalmol 2017;40(8):696-705.
https://doi.org/10.1016/j.jfo.2017.04.003
PMid:28916115
|
|
|
61 Markomichelakis NN, Aissopou EK, Maselos
S, Tugal-Tutkun I, Sfikakis PP. Biologic treatment options for retinal
neovascularization in behçet's disease. Ocul Immunol Inflamm
2019;27(1):51-57.
https://doi.org/10.1080/09273948.2017.1332228
PMid:28700280
|
|
|
62 Scott AW. Ophthalmic manifestations of
sickle cell disease. South Med J 2016;109(9):542-548.
https://doi.org/10.14423/SMJ.0000000000000525
PMid:27598358
|
|
|
63 Dyer G, Rohl A, Shaikh S. Ocular
sarcoidosis limited to retinal vascular ischemia and neovascularization.
Cureus 2016;8(10):e839.
https://doi.org/10.7759/cureus.839
PMid:27928517 PMCid:PMC5119952
|
|
|
64 Errera MH, Pratas A, Goldschmidt P,
Sedira N, Sahel JA, Benesty J. Eales' disease. J Fr Ophtalmol 2016;39(5):474-482.
https://doi.org/10.1016/j.jfo.2016.03.001
PMid:27185661
|
|
|
65 Dammacco, R. Systemic lupus
erythematosus and ocular involvement: an overview. Clin Exp Med
2018;18(2):135-149.
https://doi.org/10.1007/s10238-017-0479-9
PMid:29243035
|
|
|
66 Liao J, He Q, Li M, Chen Y, Liu Y, Wang
J. LncRNA MIAT: myocardial infarction associated and more. Gene 2016;578(2):158-161.
https://doi.org/10.1016/j.gene.2015.12.032
PMid:26707210
|
|
|
67 Li F, Wen XY, Zhang H, Fan XQ. Novel insights
into the role of long noncoding RNA in ocular diseases. Int J Mol Sci
2016;17(4):478.
https://doi.org/10.3390/ijms17040478
PMid:27043545 PMCid:PMC4848934
|
|
|
68 Yan B, Yao J, Liu JY, Li XM, Wang XQ, Li
YJ, Tao ZF, Song YC, Chen Q, Jiang Q. LncRNA-MIAT regulates microvascular
dysfunction by functioning as a competing endogenous RNA. Circ Res
2015;116(7): 1143-1156.
https://doi.org/10.1161/CIRCRESAHA.116.305510
PMid:25587098
|
|
|
69 Jaé N, Dimmeler S. Long noncoding RNAs
in diabetic retinopathy. Circ Res 2015;116(7):1104-1106.
https://doi.org/10.1161/CIRCRESAHA.115.306051
PMid:25814678
|
|
|
70 Hutchinson JN, Ensminger AW, Clemson CM,
Lynch CR, Lawrence JB, Chess A. A screen for nuclear transcripts identifies
two linked noncoding RNAs associated with SC35 splicing domains. BMC Genomics
2007;8:39.
https://doi.org/10.1186/1471-2164-8-39
PMid:17270048 PMCid:PMC1800850
|
|
|
71 Wilusz JE. Long noncoding RNAs:
re-writing dogmas of RNA processing and stability. Biochim Biophys Acta
2016;1859(1):128-138.
https://doi.org/10.1016/j.bbagrm.2015.06.003
PMid:26073320 PMCid:PMC4676738
|
|
|
72 Wu Q, Meng WY, Jie Y, Zhao H. LncRNA MALAT1
induces colon cancer development by regulating miR-129-5p/HMGB1 Axis. J Cell
Physiol 2018;233(9):6750-6757.
https://doi.org/10.1002/jcp.26383
PMid:29226325
|
|
|
73 Zhang XJ, Hamblin MH, Yin KJ. The long
noncoding RNA Malat1: its physiological and pathophysiological functions. RNA
Biol 2017;14(12):1705-1714.
https://doi.org/10.1080/15476286.2017.1358347
PMid:28837398 PMCid:PMC5731810
|
|
|
74 Zhang B, Arun G, Mao YS, Lazar Z, Hung
G, Bhattacharjee G, Xiao XK, Booth CJ, Wu J, Zhang CL, Spector DL. The lncRNA
Malat1 is dispensable for mouse development but its transcription plays a cis-regulatory
role in the adult. Cell Rep 2012;2(1):111-123.
https://doi.org/10.1016/j.celrep.2012.06.003
PMid:22840402 PMCid:PMC3408587
|
|
|
75 Tang D, Yang Z, Long F, Luo L, Yang B,
Zhu R, Sang X, Cao G. Inhibition of MALAT1 reduces tumor growth and
metastasis and promotes drug sensitivity in colorectal cancer. Cell Signal
2019;57: 21-28.
https://doi.org/10.1016/j.cellsig.2019.01.013
PMid:30716387
|
|
|
76 Gutschner T, Hämmerle M, Diederichs S.
MALAT1: a paradigm for long noncoding RNA function in cancer. J Mol Med
2013;91(7): 791-801.
https://doi.org/10.1007/s00109-013-1028-y
PMid:23529762
|
|
|
77 Zhang XJ, Tang XL, Liu K, Hamblin MH,
Yin KJ. Long noncoding RNA malat1 regulates cerebrovascular pathologies in
ischemic stroke. J Neurosci 2017;37(7):1797-1806.
https://doi.org/10.1523/JNEUROSCI.3389-16.2017
PMid:28093478 PMCid:PMC5320610
|
|
|
78 Zhang XJ, Tang XL, Hamblin M, Yin KJ. Long
non-coding RNA malat1 regulates angiogenesis in hindlimb ischemia. Int J Mol
Sci 2018;19(6):1723.
https://doi.org/10.3390/ijms19061723
PMid:29891768 PMCid:PMC6032369
|
|
|
79 Liu JY, Yao J, Li XM, Song YC, Wang XQ,
Li YJ, Yan B, Jiang Q. Pathogenic role of lncRNA-MALAT1 in endothelial cell
dysfunction in diabetes mellitus. Cell Death Dis 2014;5:e1506.
https://doi.org/10.1038/cddis.2014.466
PMid:25356875 PMCid:PMC4649539
|
|
|
80 Yan B, Tao ZF, Li XM, Zhang H, Yao J,
Jiang Q. Aberrant expression of long noncoding RNAs in early diabetic
retinopathy. Invest Ophthalmol Vis Sci 2014;55(2):941-951.
https://doi.org/10.1167/iovs.13-13221
PMid:24436191
|
|
|
81 Liu JY, Yao J, Li XM, Song YC, Wang XQ,
Li YJ, Yan B, Jiang Q. Pathogenic role of lncRNA-MALAT1 in endothelial cell
dysfunction in diabetes mellitus. Cell Death Dis 2014;5:e1506.
https://doi.org/10.1038/cddis.2014.466
PMid:25356875 PMCid:PMC4649539
|
|
|
82 Michalik KM, You XT, Manavski Y,
Doddaballapur A, Zörnig M, Braun T, John D, Ponomareva Y, Chen W, Uchida S,
Boon RA, Dimmeler S. Long noncoding RNA MALAT1 regulates endothelial cell
function and vessel growth. Circ Res 2014;114(9):1389-1397.
https://doi.org/10.1161/CIRCRESAHA.114.303265
PMid:24602777
|
|